Our Research
The key theme of research at the CRUK Manchester Institute is cellular heterogeneity in cancer: from mechanism to clinic. Our research spans the whole spectrum of cancer research including, but not limited to, tumour-host interactions, microenvironment, biophysical regulation of tumour function, and genetic and non-genetic drivers of tumour evolution and response to therapy. We are well-renowned for excellent multi-disciplinary research, working in close collaboration with The Christie NHS Foundation Trust on translational research and the development of novel therapeutic approaches. Our integration and collaboration provide an exceptional environment in which to pursue basic, translational, and clinical research programmes. Our research programmes are strengthened by access to the MCRC Tissue Biobank which contains over 150,000 samples.
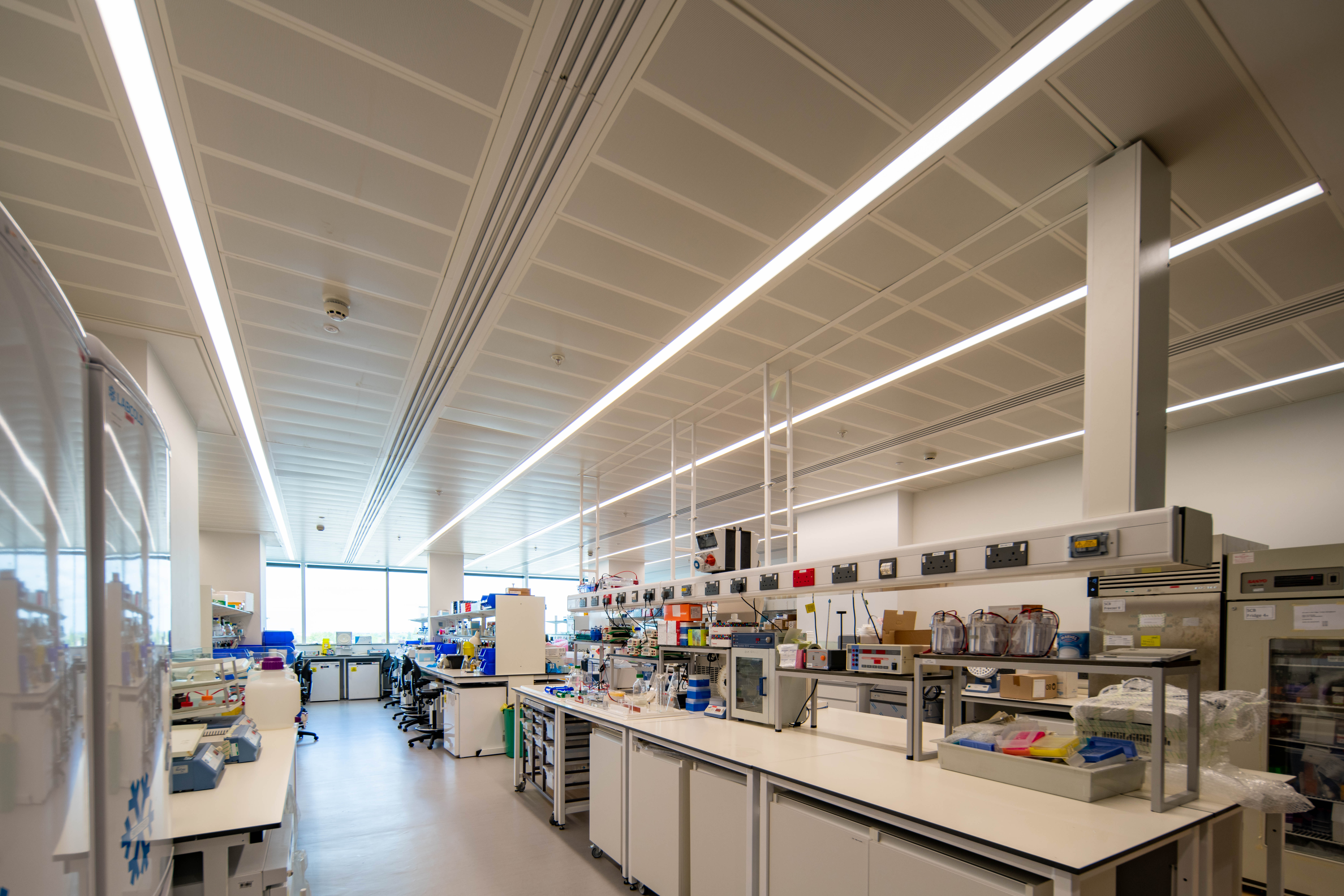
The Institute is core funded by Cancer Research UK, the largest independent cancer research organisation in the world, and our research is guided by Cancer Research UK’s Research Strategy. Our aim is to gain a greater understanding of the fundamental basis of how cancer develops and apply that knowledge to develop new diagnostic tests, treatments, and ways to predict patient outcomes.
We have extensive cutting-edge research facilities and our core services and technologies contain state-of-the-art equipment to support research projects across the Institute. Find out more on our Services and Technology page. The facilities also provide support for processing of clinical samples from early phase trial patients from The Christie and other hospitals in Greater Manchester, with lab spaces being managed to GLP compliance where required.
A number of groups have active collaborations with industry to develop, assess and deliver novel therapies. Institute groups are involved in a number of clinical trials at hospitals across the country. Our links with industry are well developed, particularly in the translational and clinical research areas. Our alliance with AstraZeneca has resulted in joint initiatives in biomarker research and training opportunities.
CRUK Manchester Institute publications are available as open access articles at Europe PubMed Central. See our Publications page or individual ORCID links on each group page.
CRUK Manchester Institute Research Groups
Director
Small Cell Lung Cancer Biology
National Biomarker Centre
Deputy Director
Translational Oncogenomics
Cancer Inflammation and Immunity
Cancer Immunosurveillance
Institute Fellows
Translational Lung Cancer Biology
Leukaemia Immunology & Transplantation
Team Leads
Cancer Biomarker Centre
Deputy Director
Bioinformatics and Biostatistics
digital Experimental Cancer Medicines Team (dECMT)